
The Simons Foundation Autism Research Initiative (SFARI) hosted its 15th science meeting April 7–9, 2019. Over 100 SFARI Investigators, collaborators and foundation staff convened to discuss new discoveries on genetic changes, molecular- and circuit-level alterations and clinical aspects of autism spectrum disorder (ASD), as well as opportunities for intervention.
- SFARI fall 2018 science meeting highlighted recent findings in autism research
- SPARK workshop discussed digital tools for phenotyping cognition and behavior in autism spectrum and other brain disorders
- SFARI workshop revisited the excitation-inhibition hypothesis of autism
- SFARI Viewer, an online platform to visualize and analyze SFARI genomic data, has launched
Keynote presentations
Recapitulating human cortical development in a dish
The meeting kicked off with a keynote address by Jürgen Knoblich, a pioneer of the methodology behind brain organoids. Discussing the applications of brain organoids to model brain development in health and disease, he argued that these 3-D cultured stem cells can recapitulate unique aspects of human cortical development that are not found in other species, thus being especially advantageous to study human-specific neurodevelopment and associated disorders1. Knoblich’s group has used brain organoids to model several disorders — including microcephaly, a phenotype associated with the autism-linked 16p11.2 duplication syndrome and other conditions — that have been difficult to reproduce in rodent systems2.
Developing algorithms to find treatments for rare diseases
The Monday night keynote presentation was given by Matthew Might, a specialist in precision medicine approaches and parent of a child with a rare genetic condition. Originally trained as a computer scientist, Might entered the field of precision medicine as the result of his personal odyssey to find the causes of his son’s disorder, which were unknown at the time. This journey led him to discover that his son was ‘patient zero’ of an enzymatic deficiency caused by a pathogenic mutation in the NGLY1 gene that prevents degradation of glycoproteins and to start research efforts to characterize the pathophysiological mechanisms of this newly discovered condition and identify potential treatments.
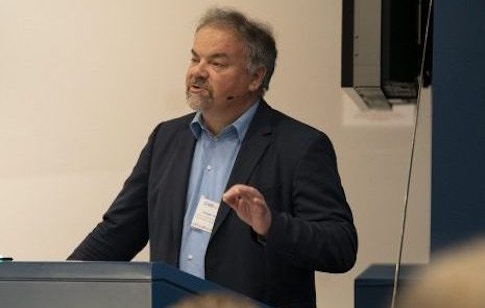
Today, Might works to help families with rare genetic disorders to find answers and, possibly, therapeutic options. To aid in this endeavor, he has also developed novel technological tools, including algorithms that can search and analyze the biomedical literature to identify and repurpose available drugs that are, in many cases, FDA approved — an approach that he speculated could bring insights to disorders such as ASD, for which no pharmacological therapeutics are currently available.
Session presentations
Fourteen investigators presented their latest research findings during four sessions on the topics of genetics; molecular mechanisms; circuits, systems, cognition and behavior; and clinical studies.
Genetics
Genetic risk factors
With over 100 genes and numerous copy number variations (CNVs) now being linked to the condition, many are the factors at play in ASD genetics. The first speaker of the genetics session, Hilary Coon, argued that the study of rare and common variation, which both contribute to ASD risk, can be combined to build polygenic risk scores and identify genetically characterized subtypes of autism. Focusing on rare variation, she also reported several de novo ASD-associated variants that she identified in the Simons Simplex Collection (SSC) thanks to a new data-analysis pipeline for whole-genome sequencing developed in collaboration with Gabor Marth.
Another speaker, Santhosh Girirajan, focused on the relationship between ASD genotype(s) and phenotype(s), an aspect of the disorder that has proven difficult to characterize. Girirajan claimed that the lack of a one-to-one genotype-to-phenotype relationship may depend on the modulatory effect of rare, likely deleterious variants in the genetic background. In support of this hypothesis, he showed that probands with 16p12.1 deletion, a CNV linked to ASD and other disorders, have additional rare variants in functionally intolerant genes, or ‘other hits,’ compared to unaffected parents who also carried the variant3. Moreover, the number of other hits correlated with the severity of cognitive impairment in probands with a number of other disease-associated CNVs (Figure 1). He concluded that the compounded effects of primary and secondary hits in the genome ultimately determines the neurodevelopmental phenotypes — a proposition that Girirajan previously referred to as the ‘two-hit genetic model’4,5.
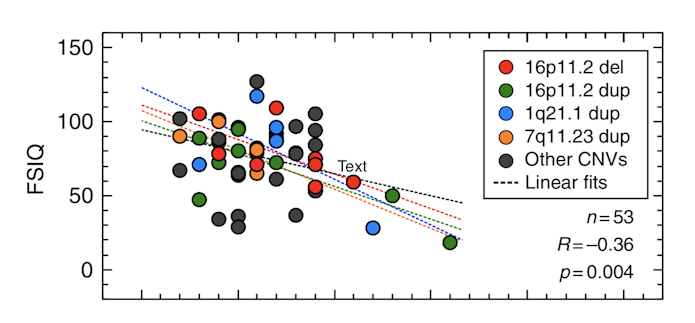
From genes to transcriptomics
In addition to identifying genetic risk factors, scientists have also started to investigate the impact of pathological genetic changes on the transcriptome. Kathryn Roeder presented findings from a collaborative study6 with Haiyuan Yu, where they reported that probands from the SSC carried de novo missense mutations that disturbed interactions between proteins at higher rates than mutations found in unaffected siblings. These mutations also selectively affected proteins that play a central role as ‘network hubs’ in the human interactome.
She also described new analytical tools that should help to achieve a better understanding of gene expression changes, such as MIND (Multi-measure INdividual Deconvolution), a technique that allows one to deconvolve gene expression signal from bulk tissue analysis and identify expression in individual cell types7. Using this methodology, Roeder and her colleagues have built gene co-expression networks for individual brain cell types and found strong correlations between the expression of ASD genes relative to non-ASD genes in immature neurons (but not in astrocytes, oligodendrocyte progenitor cells, oligodendrocytes or mature neurons) — a result consistent with evidence showing that ASD-associated changes may occur in the early stages of brain development8.
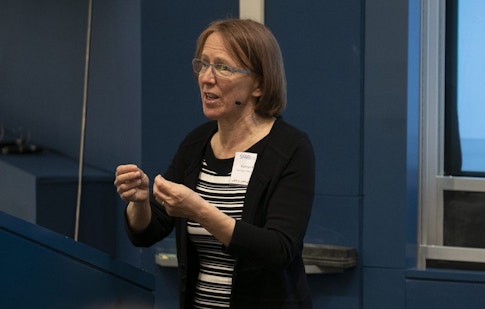
Moving onto a mechanistic description of the disorder, the final speaker of this session, Benjamin Blencowe, discussed his research on nSR100/SRRM4, a neuronal-specific splicing regulator that controls neural microexons — small 3-27 nucleotide-long exons involved in neurogenesis and protein-protein interaction. Blencowe had previously found that neural microexons are dysregulated in ASD and that decreased nSR100/SRRM4 expression correlates with a reduction in microexon splicing in postmortem brain samples from individuals with ASD9.
His most recent work follows up these findings by investigating whether dysregulation of nSR100/SRRM4 plays a causal role in ASD-related pathology by studying a mouse model lacking one copy of the nSR100/SRRM4 gene. He found that these mutant mice displayed behavioral and cellular phenotypes relevant to ASD and that nSR100/Srrm4 was regulated by neuronal activity10. He is now working to assess whether modulation of nSR100/SRRM4 and microexon activity represents a potential therapeutic strategy for the treatment of ASD.
Molecular mechanisms
The session on molecular mechanisms focused on aspects of cell physiology that are affected by ASD-linked genetic mutations and discussed findings from gene therapy studies that are currently being conducted for genetic subtypes of ASD due to loss-of-function of SCN2A or UBE3A.
Signaling molecules
The first speaker, Kassandra Ori-McKenney, presented her research on kinases that regulate DYRK1A, a high-confidence autism risk gene that has been also implicated in learning disabilities in Down syndrome. Using the fly as a model organism, she showed that Dyrk1a plays a role in regulating the cell cytoskeleton and the length of neuronal dendrites11. She is now working to identify downstream targets of DYRK1A and plans to conduct similar studies in the mammalian brain in the near future.
While the majority of SFARI-funded research focuses on neurons, non-neuronal cells, such as astrocytes, also appear to play a role in ASD pathophysiology. The next speaker, Cagla Eroglu, argued that cell adhesion molecules that regulate the communication between astrocytes and neurons, such as those in the neurexin/neuroligin family, are crucial to determine astrocyte morphogenesis and synapse number and function. Astrocytes have complex structures that can communicate to hundreds of thousands of synapses at once. If neuroligins (NL1, NL2, NL3) are removed from astrocytes, however, the structure of astrocytes becomes simpler12. Furthermore, Eroglu’s studies have shown that when NL2 is selectively deleted from astrocytes in mice, cortical excitatory synapse numbers are reduced and changes in excitatory/inhibitory balance are observed. As many of these cellular phenotypes are found in ASD, she hypothesized that the pathways regulating bidirectional communication between neurons and astrocytes may be affected in disorders such as ASD. She plans to test this hypothesis by screening ASD-associated genes that are highly expressed in astrocytes, a project that is supported by her SFARI Pilot Award.

Protein interaction networks
Another speaker in this session, Kasper Lage, discussed the importance of studying how genes interact at the protein level to better understand the impact of genetic insults on protein-protein interactions and find therapeutics that can ‘un-perturb’ those disrupted protein networks. He argued that although genetics has been giving important clues about biology, the underlying protein interaction networks, and their function, remain uncharted territory.
The Lage laboratory has developed many computational resources13-15, some of which were showcased during the meeting’s demo session. Lage’s research has focused on a variety of diseases and conditions, spanning from cancer to heart disease to schizophrenia; through a SFARI Research Award, he is now studying biological networks that are specifically disturbed in ASD. To this end, he selected proteins that are encoded by ASD risk genes identified in a recent study16 and is planning to find their interaction partners in billions of neurons cultured in vitro — a feat made possible by the large-scale production of stem-cell-derived neurons at the Stanley Center for Psychiatric Research (Broad Institute).
Gene therapy approaches
• Boosting RNA
The advent of CRISPR-integrase based technology has ushered in new opportunities for intervention for many genetic disorders, including those caused by gene haploinsufficiency, the lack of one functional gene copy. Approaches such as CRISPR-mediated activation (CRISPRa), which involves adding a transcriptional activator that increases RNA expression, may provide a therapeutic option for haploinsufficient conditions by boosting RNA expression of the functional gene copy.
Nadav Ahituv has been using this methodology to study a potential treatment for severe forms of obesity associated with SIM1 or MC4R haploinsufficiency. In his presentation, he demonstrated that a single administration of a CRISPRa-recombinant adeno-associated virus (AAV) vector rescued the pathological phenotype in mouse models of this disorder, by increasing Sim1 or Mc4r expression and restoring body weight to wild-type levels1. Of note, these effects lasted for months.
With funding from a SFARI Explorer Award and a subsequent Director Award (in collaboration with Kevin Bender), Ahituv is now assessing whether a CRISPRa gene therapy approach can rescue Scn2a haploinsufficiency in mice. One of the first ASD risk genes identified from the SSC, SCN2A remains one of the genes that has the strongest evidence for association with ASD18 and that has been extensively studied at the physiological and cellular levels, both in in vitro and in vivo models19. Leveraging these studies, Ahituv and colleagues will assess whether AAV CRISPRa administration rescues some of the cellular and behavioral phenotypes that have been recently observed in Scn2a haploinsufficient mice20.
• Waking up a silenced allele
Another example of an ASD-related genetic condition with one disrupted gene copy is Angelman syndrome. In this condition, the maternal copy of the UBE3A gene is deleted or functionally inactive. But because the paternal copy of UBE3A is normally silenced in mature neurons due to the action of an antisense transcript, individuals with loss of function of the maternal copy are left with virtually no UBE3A. Mark Zylka reasoned that if the paternal gene copy could be reactivated, the Angelman phenotype may potentially be rescued.
“We have been learning increasingly more about what may be ‘broken’ in the autism brain and the question is: Can it be fixed?” asked Zylka.

To test his hypothesis, Zylka used a CRISPR inactivation approach to block the expression of the antisense transcript that normally silences the paternal allele and, hence, reactivates Ube3a expression. He is testing such an approach both in neurons derived from induced pluripotent stem cells and in a mouse model of Angelman syndrome (Figure 2) with the ultimate goal to identify a treatment strategy for this condition.
Circuits, systems, cognition and behavior
The session on circuits, systems, cognition and behavior discussed SFARI-funded research that uses animal models to study neural circuits affected in ASD.

Gina Turrigiano presented results from her studies on the role of SHANK3 in regulating homeostastic plasticity — a compensatory mechanism that stabilizes neuronal activity after perturbation. SHANK3 is one of the genes in the 22q13 chromosomal region that is deleted in Phelan-McDermid syndrome, a disorder that is frequently associated with ASD and intellectual disability. Imbalances in neuronal excitation and inhibition (E/I) are important in the context of ASD because they are thought to be a key biological substrate of certain ASD phenotypes, such as seizures21. Because Shank3 is enriched in postsynaptic densities of excitatory synapses, Turrigiano hypothesized that changes in the Shank3 gene may lead to failure in homeostatic plasticity, which, in turn, may result in circuit hypo- or hyperactivity.
To test this hypothesis, she used a sparse knockdown approach in cultured cortical neurons to show that Shank3 is required for two major forms of homeostatic plasticity: synaptic scaling and intrinsic homeostatic plasticity22. Interestingly, both forms of plasticity could be rescued by treatment with lithium, a drug that has previously shown some efficacy in two case studies of patients with SHANK3 mutations and ASD23. Turrigiano also demonstrated that Shank3 loss in vivo severely compromised the ability of visual cortical circuits to recover from perturbations to sensory drive, thus suggesting that loss of homeostatic compensation may underlie circuit dysfunction in Phelan-McDermid syndrome and, potentially, other ASD subtypes.
Next, Loren Frank discussed his studies using Fmr1 knockout rats to investigate the neural circuits and related behavioral deficits associated with fragile X syndrome, the most commonly identified single-gene cause of ASD and intellectual disability. He showed that Fmr1 mutant rats performed similarly to wildtype in an acoustic ‘prepulse inhibition’ task24, an experimental paradigm that refers to the reduction in the response to a startling sound when the startle is preceded by a weak prestimulus, or prepulse. This behavioral measure has been used to study abnormalities of sensorimotor gating in schizophrenia as well as in ASD.
Frank is now examining the behavior of Fmr1 knockout rats in other assays, including tasks assessing spatial memory and probing the underlying neuroanatomical circuits. In his presentation, he reported preliminary findings suggesting that loss of Fmr1 may cause the rats to develop alternative behavioral strategies compared to wildtype rats, thus raising the possibility that Fmr1 knockout rats might use their hippocampus differently and, more broadly, that insights about which circuits support specific behaviors in wildtype animals may need to be reexamined in the context of disease models.
Clinical studies
The clinical session included discussions about a potentially novel approach to treat cognitive deficits in individuals with ASD, as well as the presentation of a digital tool aimed at improving autism screening and diagnosis.
Sleep spindles
Many individuals with ASD report sleep disturbances, yet the role of sleep in autism remains little understood. Dara Manoach, who studies sleep and cognition in neurodevelopmental disorders such as schizophrenia, argued that sleep has profound beneficial effects on cognition by helping consolidate and enhance learning occurred during the day25 in typically developing individuals, but not in those with schizophrenia26. Individuals with schizophrenia also exhibited abnormalities in the activity of sleep spindles — thalamocortical oscillations of stage 2 nonrapid eye movement (NREM) sleep that are known to play a role in memory consolidation27.
Thanks to funding support from the Simons Center for the Social Brain, Manoach is now expanding her research on spindle activity to ASD. As mouse and human studies demonstrate that spindle activity can be modulated to improve memory28, the hope is that sleep spindles may be one potentially treatable mechanism of cognitive dysfunction in ASD.
Screening and monitoring at scale
One challenge in the autism clinical community has been to provide accurate and timely diagnoses, so that behavioral interventions can start when the brain is most plastic. However, some children still receive a diagnosis later in childhood, argued Guillermo Sapiro. To accelerate screening and monitoring of the increasing number of individuals with neurodevelopmental disorders worldwide and make these tools available to countries where access to health care may be prohibitive, Sapiro proposed leveraging digital phenotyping technologies for assessing autism symptoms outside the clinic.
To this end, he and his collaborators have developed computer vison and machine learning algorithms that analyze children’s facial expressions and emotions while they watch videos eliciting ASD symptoms on a smartphone. In his presentation, Sapiro discussed initial results from this research, showing that, relative to their unaffected peers, children with ASD exhibited atypical postural control, with increased difficulty maintaining the midline position of the head29 (Figure 3). Moving beyond vision-based data, Sapiro now plans to collect information from other sensory modalities such as audio and touch, with the goal to provide a composite, multimodal approach to understand the diverse complexity of ASD phenotype(s).
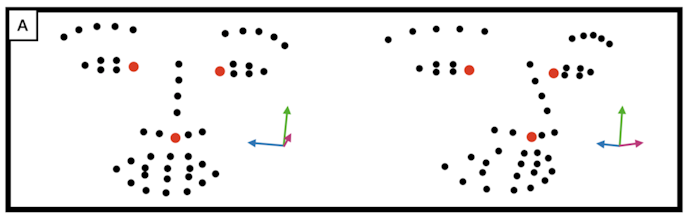
While digital phenotyping will help to gather large amounts of data and yield valuable insights into the disorder, many questions pertaining to its application for clinical assessment remain to be discussed. For instance, how will the results of such screening tools be delivered? And how will families receive information about pertinent next steps, including referrals to confirm any such findings with medical professionals?
Panel presentations
In addition to the session presentations described above, the meeting also convened a number of short presentations and a panel discussion centered around the topic of ‘animal behavior’ and the development of novel methodologies to improve behavioral analysis in rodents.
Animal-based research has been, and still is, instrumental in uncovering fundamental aspects of the brain and behavior, as well as disease states, that are relevant for humans. By experimentally manipulating animal behavior (e.g., via a genetic or neural change, or drug administration) and assessing the effects of these manipulations under controlled conditions, scientists can test hypotheses about the underlying biological mechanisms. Though animal behavior provides a yardstick to gauge treatment effects, assessing changes objectively and reliably in experimental animals has proven challenging. Animal behavior has been traditionally annotated manually, a practice that is time consuming and prone to bias. Furthermore, because human experimenters may record only coarse instances of behavior and typically have a priori knowledge of those behaviors, subtler differences may go undetected and new, unanticipated patterns may be difficult to uncover.
The panel, which featured Ted Abel, David Anderson, Rui Costa and Sandeep Robert Datta, discussed key questions in the field of rodent behaviors that are relevant to ASD research, including describing recent efforts to develop automated and data-driven methodologies that can measure behavior objectively and, hence, overcome some of the limitations of traditional behavioral experiments.
Classifying social behavior
One of the methodologies presented at the panel was the ‘Mouse Action Recognition system’ (MARS)29. Developed by Anderson in collaboration with Pietro Perona, MARS uses a combination of video tracking and supervised machine learning algorithms to identify and classify different types of social behaviors (e.g., sniffing, mounting, aggression/attacking) in freely interacting mice (Video 1). Anderson used it to assess social behavior in BTBR mice, a mouse strain characterized by low sociality29. He is now conducting additional studies using MARS to examine social behavior in mice carrying mutations in autism risk genes, including CHD8, CUL3 and NLGN3.
MARS can be combined with an interface to track and visualize neural data in real time called the Behavior Ensemble and Neural Trajectory Observatory (BENTO). Together, these tools can be used to correlate the animal’s behavioral data with the associated neural activity on a frame-by-frame basis, but also to do the reverse: extract neural signatures that reveal patterns of behavior previously unknown to the experimenter.
Clusters and modules of motor behavior
Another topic discussed was the analysis of mouse motor behavior. Costa presented an analytical approach that relies on an unsupervised machine learning algorithm to deconstruct self-paced mouse movements into clusters, which can then be correlated with underlying neural activity30. Using this methodology, Costa demonstrated that neurons of the mouse striatum encode actions in a spatially and temporally structured way, so that ensembles of neurons underlying similar actions were spatially contiguous and temporally correlated. Costa is now working to expand this approach to investigate changes in motor behavior and associated neural activity in mouse models of autism, including 16p11.2 deletion. One goal of Costa’s research is to leverage these methodologies to learn more about the neural circuits underlying repetitive behaviors and stereotypies that are typical of ASD.
The last methodology presented at the panel was ‘Motion Sequencing’ (Moseq)31, a technology that combines 3-D machine vision with a data-driven unsupervised machine learning algorithm to dissect and extract individual modules of mouse behavior. Datta, who developed this tool, explained that the idea of Moseq was inspired by the ethological view that “all animal behavior, including that of mice, is composed out of a series of identifiable modules […] out of which the brain can compose a complete panoply of actions.” These modules can be thought of as units of stereotyped motifs, or ‘syllables,’ that are streamed together in time to produce complex meaningful behaviors (Video 2).
“Because we believe that these behavioral models reflect mouse body language, we refer to them as ‘syllables’ and the statistics that govern their sequence over time as a ‘behavioral grammar,’” Datta said.
This methodology can be used to characterize behavior in ASD mouse models at a fine-grained level of analysis and objectively capture changes to treatment, a research endeavor that Datta is conducting in 16p11.2 deletion mice. Thanks to a collaboration with the laboratory of Bernardo Sabatini, Moseq is also now compatible with software for recording neural data32; new applications of this tool to assess behavior in rat models of ASD are also being considered in partnership with Peter Kind.
Challenges and opportunities
The panelists addressed the technical issues of novel technologies and broader questions in the field. Abel argued that, alongside providing a deeper characterization of phenotype, one advantage offered by these novel technologies is the possibility to record, archive and share behavioral data.
Many challenges remain open. Autism research typically uses adult animals as model systems; Abel highlighted the importance of developing methodologies that can annotate behaviors specific to developing animals. The issue of animal systems other than rodents was also raised. Nonhuman primate models, which are evolutionarily closer to humans, may provide better insight into homologous behaviors. Yet the question remains: What behaviors, in mice or nonhuman primates, would be most relevant to study human conditions and ASD in particular? What would make a good preclinical model?
The panel concluded with a few snippets of advice to scientists interested in pursuing animal research. “Get a computational person in the lab,” said Anderson, who encouraged principal investigators to hire researchers with expertise in multidimensional data and machine learning to help with the development of advanced technologies to analyze animal behavior. “Think about controls,” Abel added, stressing the importance of data reliability; and finally, Costa reminded the field needs standards to measure behavior systematically and reliably across labs.
Demo presentations
In addition to speaker presentations, the meeting hosted demo presentations of computational tools that SFARI-funded scientists and staff from the Simons Foundation Flatiron Institute have developed to analyze genomic, proteomic and other types of data relevant to autism research.
Presenters included SFARI Investigator Ivan Iossifov, who illustrated the Genotypes and Phenotypes in Families platform, a user-friendly interface to peruse genetic and phenotypic data from the SSC, Simons Searchlight (formerly Simons Variation in Individuals Project) and SPARK (Simons Foundation Powering Autism Research for Knowledge).
Alistair Ward gave a demonstration of the recently launched SFARI Viewer, an interactive platform developed by Frameshift Genomics in collaboration with SFARI Investigator Gabor Marth that provides visual summaries of genetic and phenotypic data from the SSC and SPARK and enables users to perform data exploration and comparison to their own data sets in real time. The developers recently hosted a SFARI webinar to demonstrate the functionalities of this tool (Video 3).
Alongside tools to analyze data from SFARI cohorts, other methodologies were also presented. Heiko Horn, a former member of the Lage laboratory discussed InWeb13, NetSig14 and GeNets15, web-based resources to build gene and protein networks.
Aaron Wong, a data scientist and project leader at the Flatiron Institute, highlighted HumanBase, an online platform that was developed in collaboration with Olga Troyanskaya. This software provides data-driven predictions about gene expression, function and protein interactions in human tissues.
All of these tools are freely available to interested scientists, with access to data from SFARI cohorts being granted to approved researchers via SFARI Base.
“Overall, it was a thoroughly enjoyable meeting,” said SFARI director Louis Reichardt. “I hope that our fellow scientists and friends found the talks and interactions as stimulating as I did and came away from the meeting having learned something new and inspiring for their own work.”
For additional information about SFARI-funded investigators and research projects, please visit: https://www.sfari.org/research/investigators.
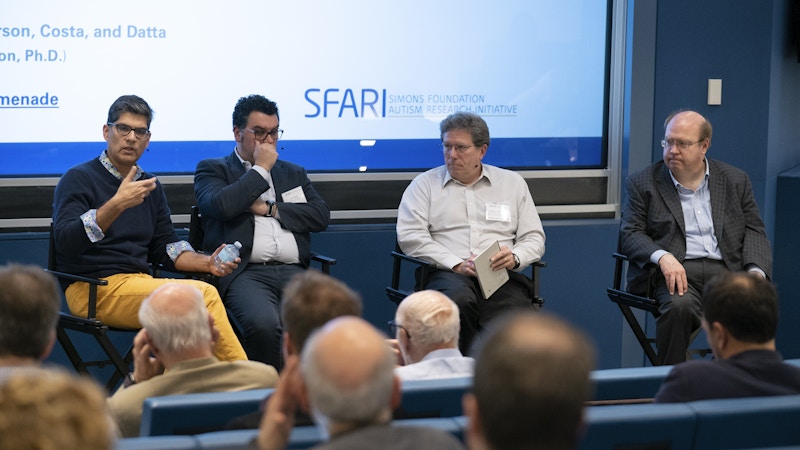
From left to right: Sandeep Robert Datta (Harvard Medical School), Rui Costa (Columbia University Medical Center), David Anderson (California Institute of Technology; Howard Hughes Medical Institute) and Ted Abel (University of Iowa) discuss new experimental approaches to measure mouse behavior during the panel session.
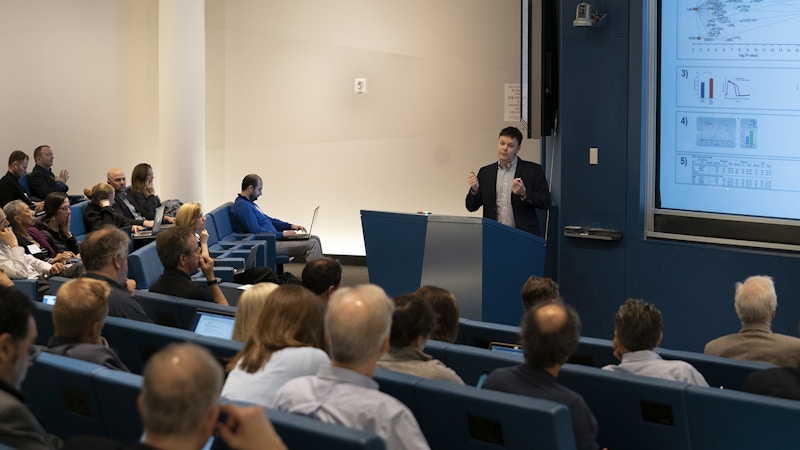
Kasper Lage of Harvard Medical School argues for an integrated bioinformatics framework that includes genetic, proteomic and neurobiological findings to advance knowledge of autism risk factors.
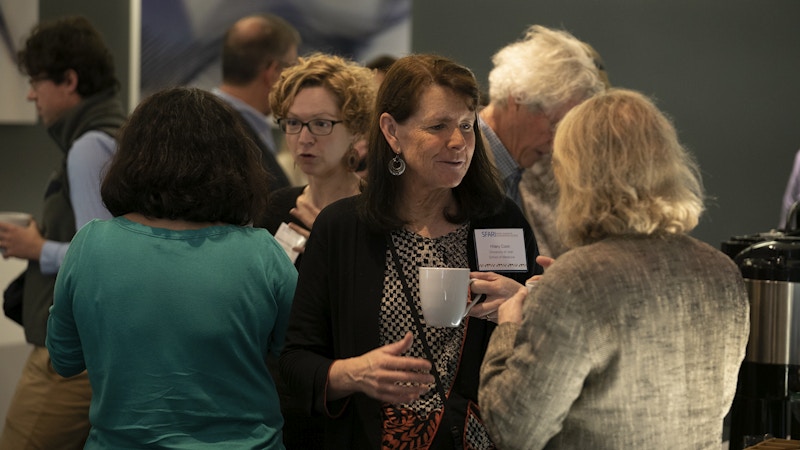
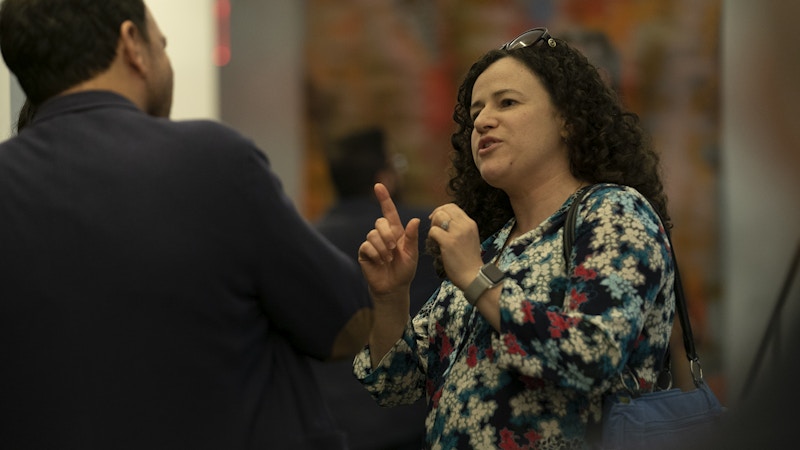
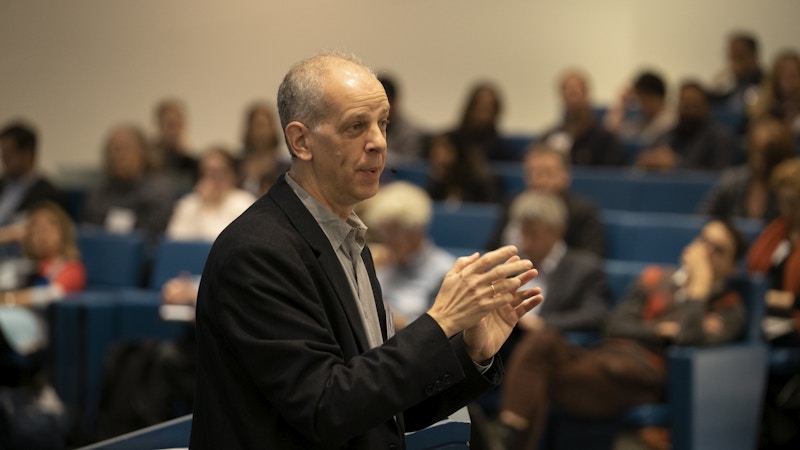
Guillermo Sapiro of Duke University discusses uses of digital technologies to phenotype behaviors relevant to autism.
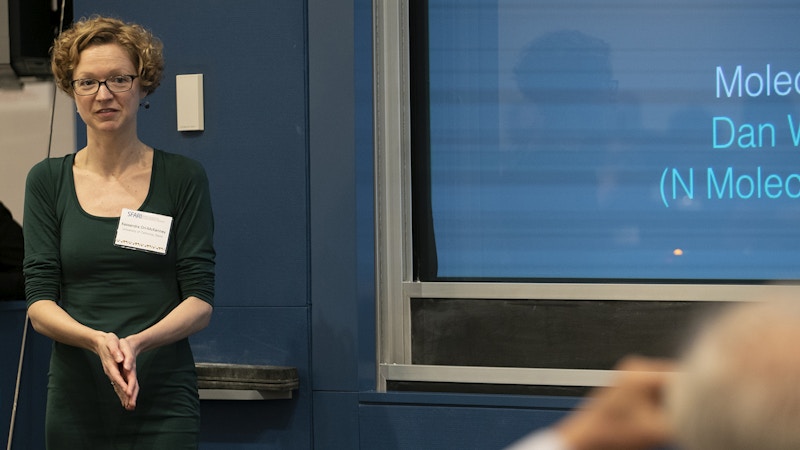
Kassandra Ori-McKenney of the University of California, Davis entertains questions from the audience about her work on signaling pathways in autism.
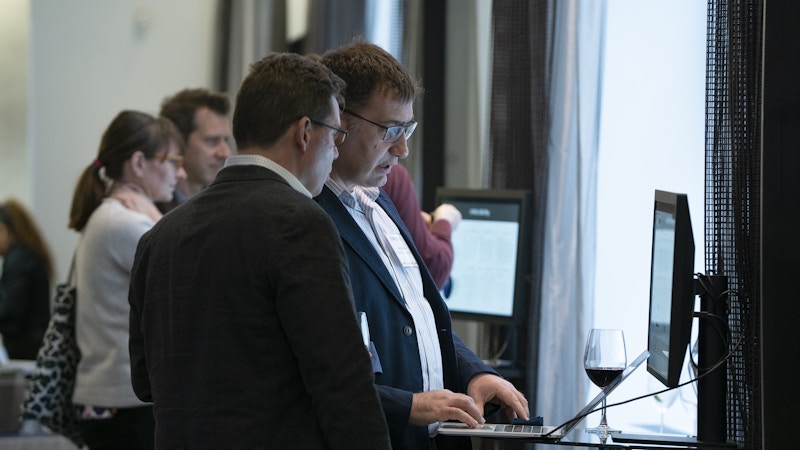
Ivan Iossifov (Cold Spring Harbor Laboratory) illustrates the functionalities of ‘Genotypes and Phenotypes in Families,’ an online platform to analyze SFARI data, during the demo session.
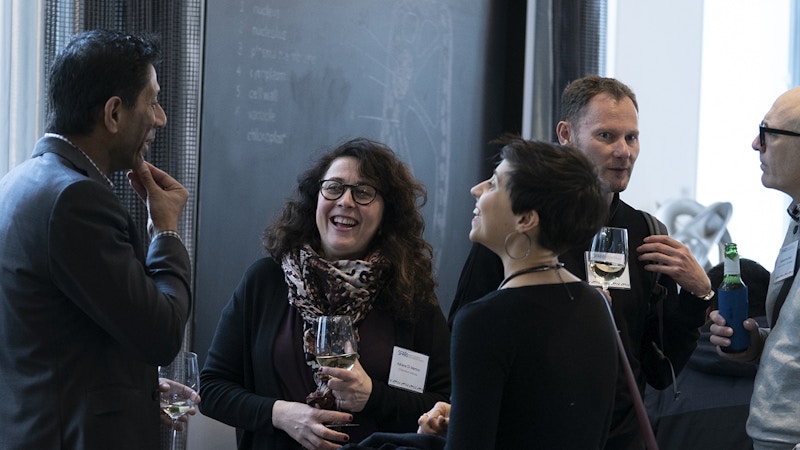
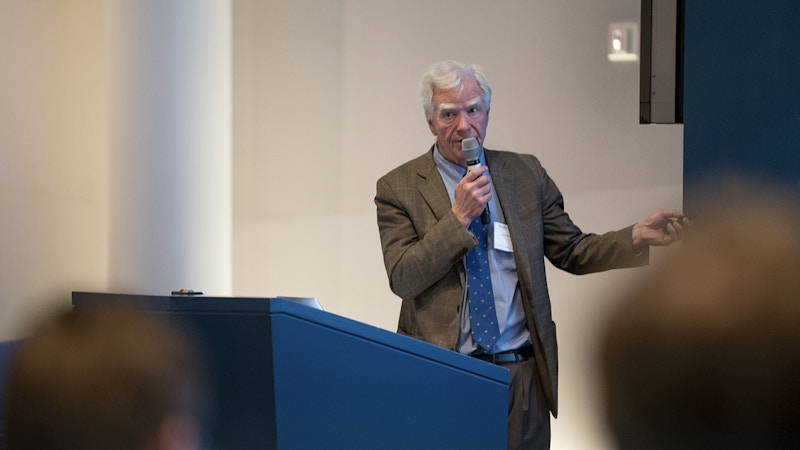
SFARI director Louis Reichardt welcomes attendees.
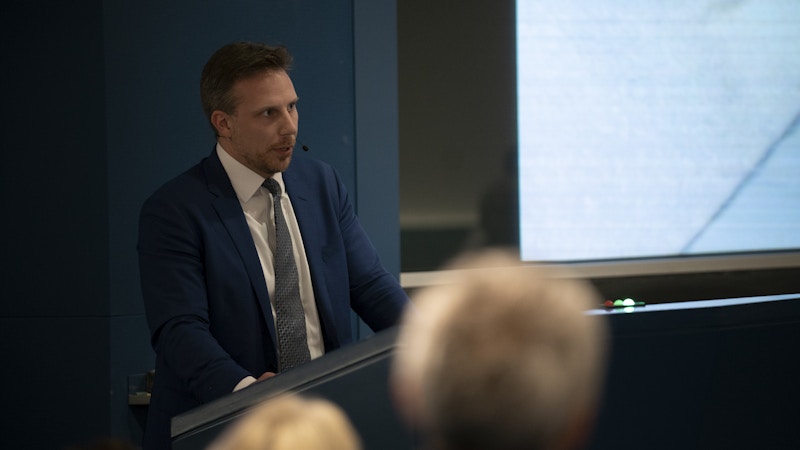
Keynote speaker Matthew Might recounts his journey into the field of precision medicine.
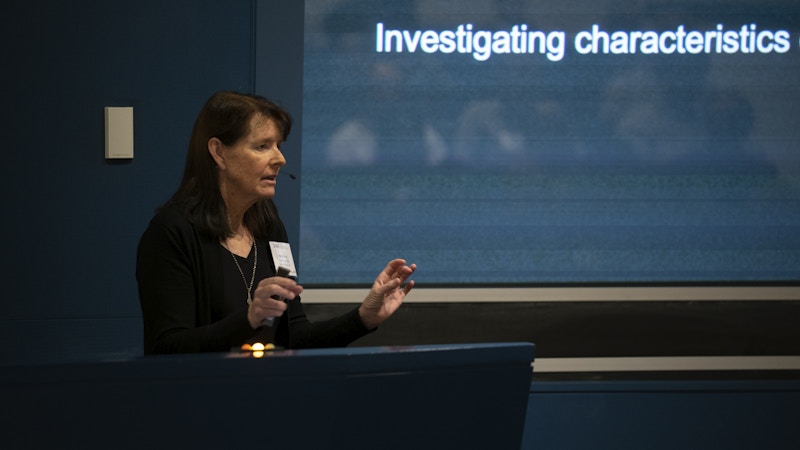
Hillary Coon of the University of Utah discusses new findings on autism genetics.
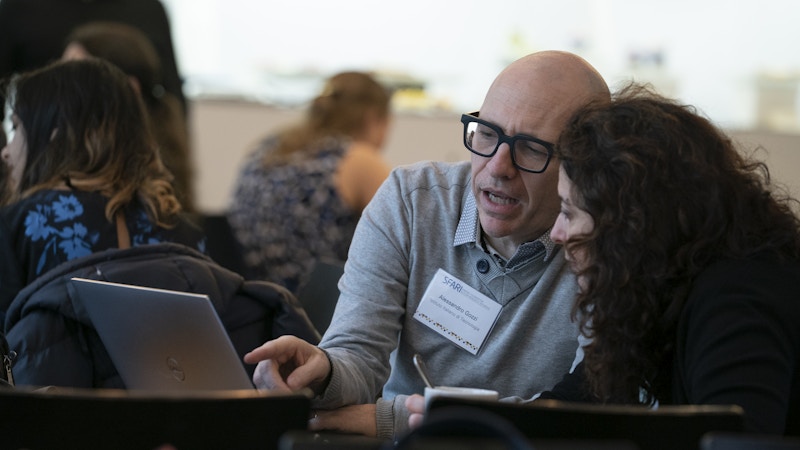
Investigators converse during coffee breaks.
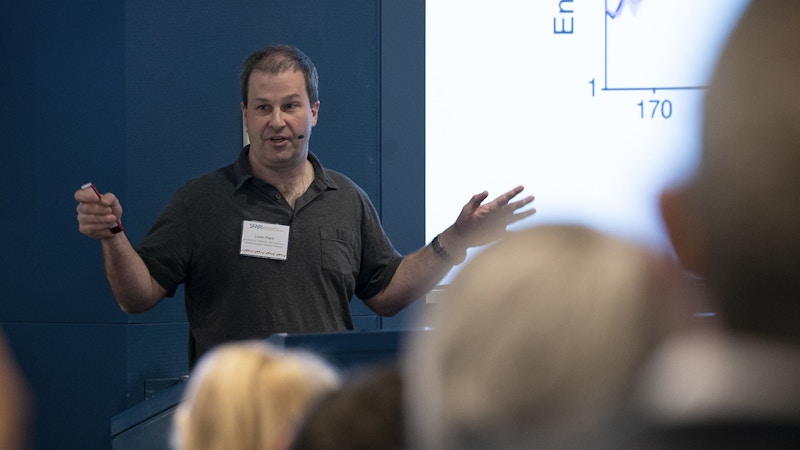
Loren Frank (University of California, San Francisco; Howard Hughes Medical Institute) argues for robust measurements of behavior in animal-based research.
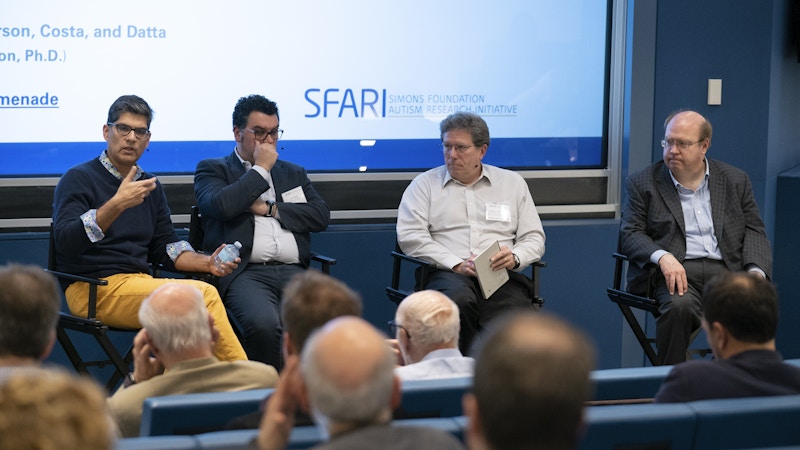
From left to right: Sandeep Robert Datta (Harvard Medical School), Rui Costa (Columbia University Medical Center), David Anderson (California Institute of Technology; Howard Hughes Medical Institute) and Ted Abel (University of Iowa) discuss new experimental approaches to measure mouse behavior during the panel session.
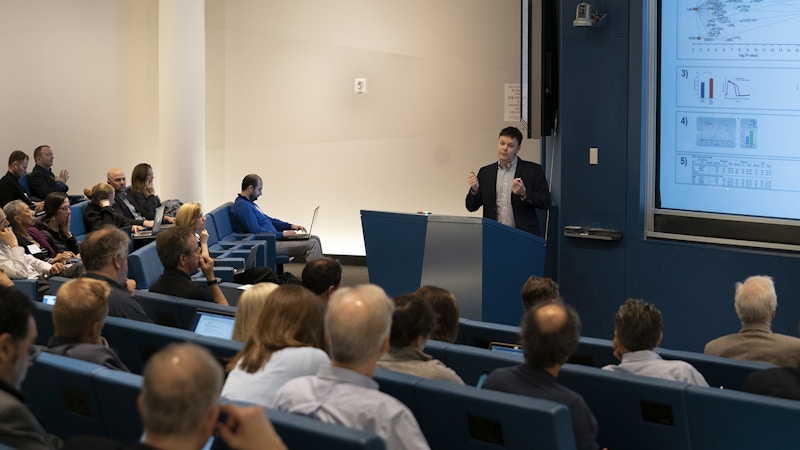
Kasper Lage of Harvard Medical School argues for an integrated bioinformatics framework that includes genetic, proteomic and neurobiological findings to advance knowledge of autism risk factors.
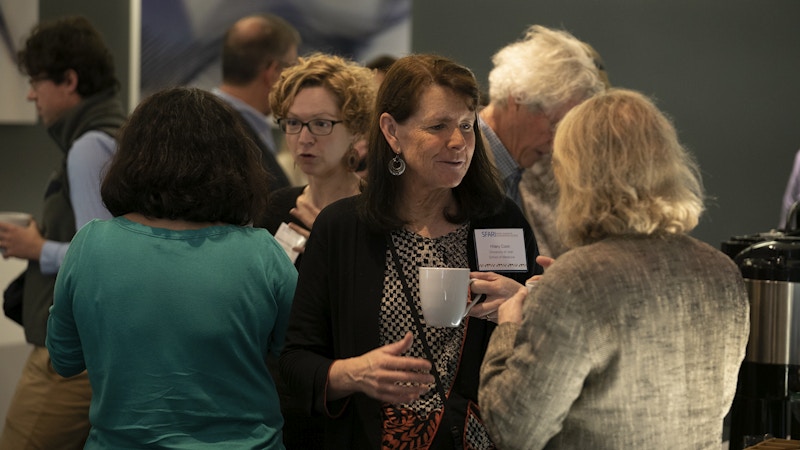
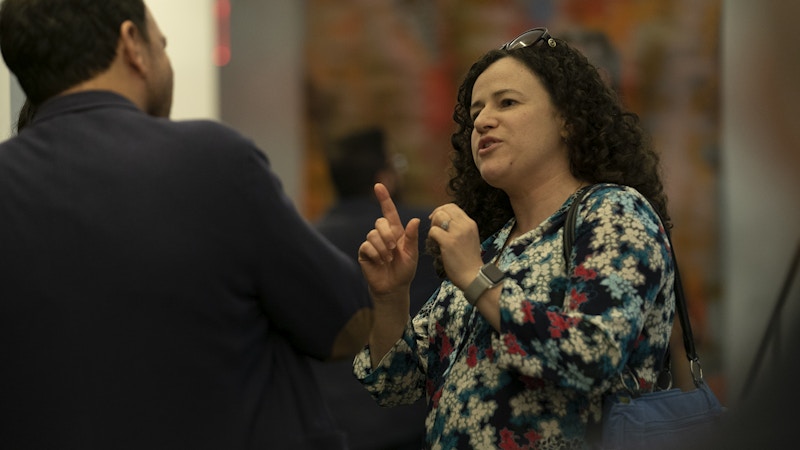
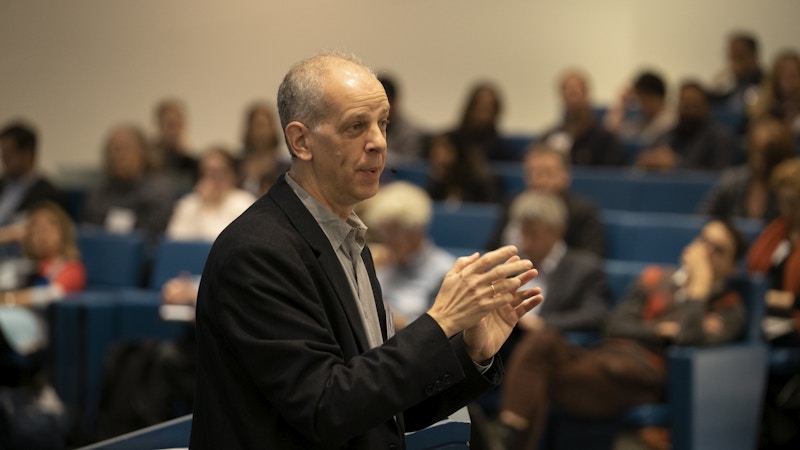
Guillermo Sapiro of Duke University discusses uses of digital technologies to phenotype behaviors relevant to autism.
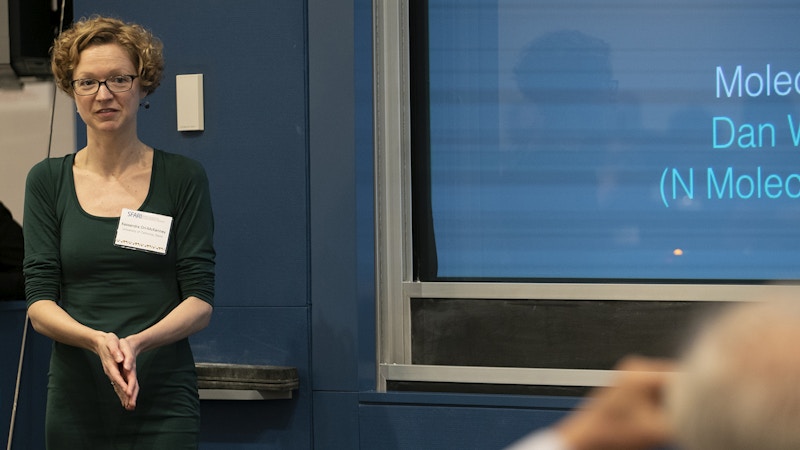
Kassandra Ori-McKenney of the University of California, Davis entertains questions from the audience about her work on signaling pathways in autism.
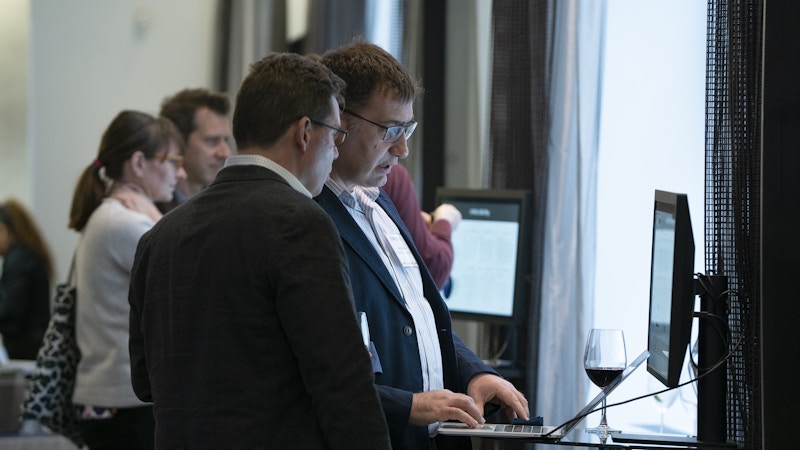
Ivan Iossifov (Cold Spring Harbor Laboratory) illustrates the functionalities of ‘Genotypes and Phenotypes in Families,’ an online platform to analyze SFARI data, during the demo session.
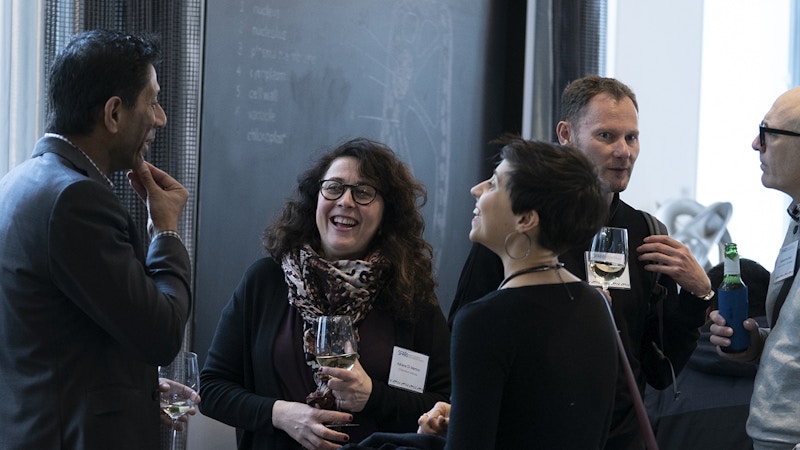
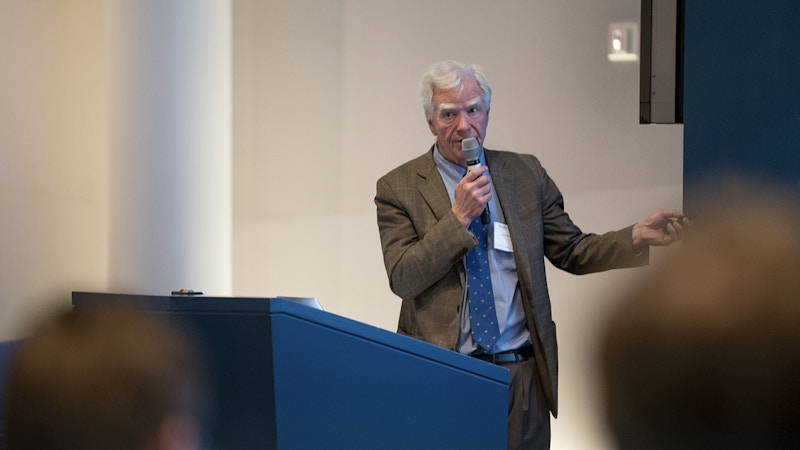
SFARI director Louis Reichardt welcomes attendees.
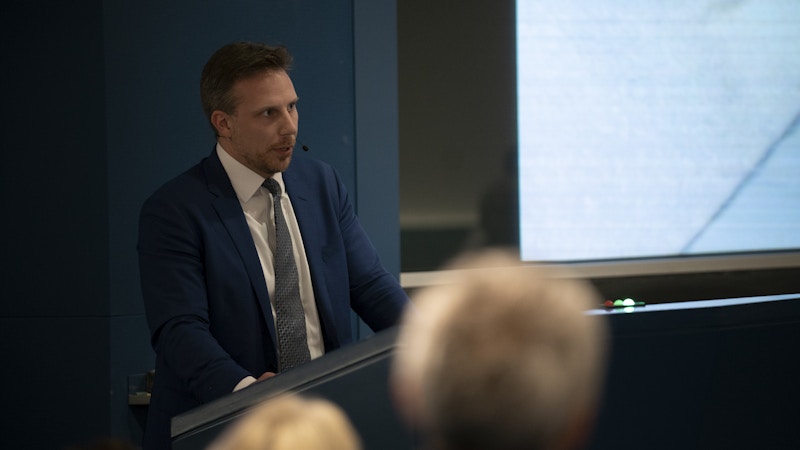
Keynote speaker Matthew Might recounts his journey into the field of precision medicine.
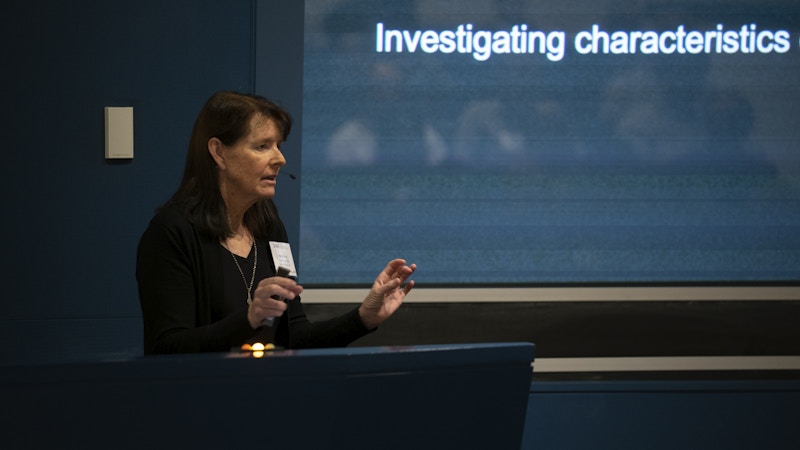
Hillary Coon of the University of Utah discusses new findings on autism genetics.
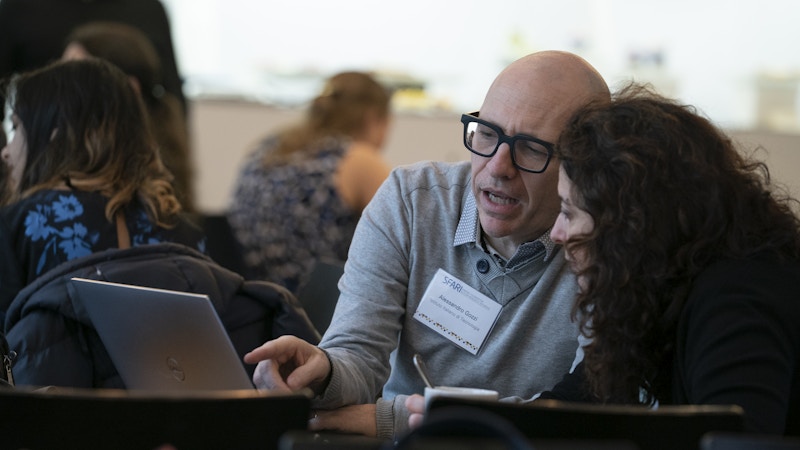
Investigators converse during coffee breaks.
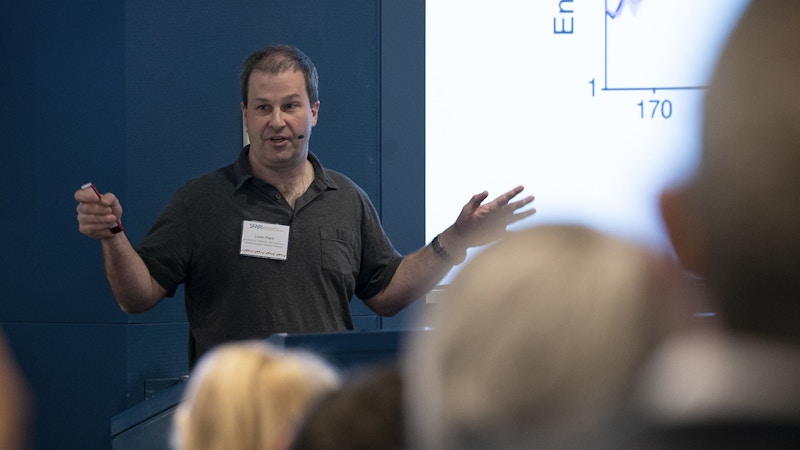
Loren Frank (University of California, San Francisco; Howard Hughes Medical Institute) argues for robust measurements of behavior in animal-based research.
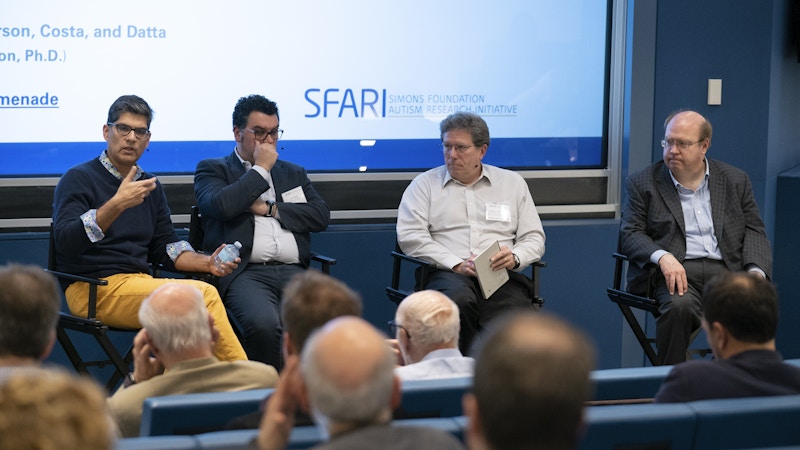
From left to right: Sandeep Robert Datta (Harvard Medical School), Rui Costa (Columbia University Medical Center), David Anderson (California Institute of Technology; Howard Hughes Medical Institute) and Ted Abel (University of Iowa) discuss new experimental approaches to measure mouse behavior during the panel session.
References
- Lancaster M.A. and Knoblich J.A. Nat. Protoc. 9, 2329-2340 (2014) PubMed
- Lancaster M.A. et al. Nature 501, 373-379 (2013) PubMed
- Pizzo L. et al. Genet. Med. 21, 816-825 (2019) PubMed
- Girirajan S. et al. Nat. Genet. 42, 203-209 (2010) PubMed
- Girirajan S. et al. N. Engl. J. Med. 367, 1321-1331 (2012) PubMed
- Chen S. et al. Nat. Genet. 50, 1032-1040 (2018) PubMed
- Wang J. et al. Bioinformatics Epub ahead of print (2019) PubMed
- Willsey A.J. et al. Cell 155, 997-1007 (2013) PubMed
- Irimia M. et al. Cell 18, 1511-1523 (2014) PubMed
- Quesnel-Vallières M. et al. Mol. Cell. 64, 1023-1034 (2016) PubMed
- Ori-McKenney K.M. et al. Neuron 90, 551-563 (2016) PubMed
- Stogsdill J.A. et al. Nature 551, 192-197 (2017) PubMed
- Li T. et al. Nat. Methods 14, 61-64 (2017) PubMed
- Li T. et al. Nat. Methods 15, 543-546 (2018) PubMed
- Horn H. et al. Nat. Methods 15, 61-66 (2018) PubMed
- Satterstrom F.K. et al. bioRxiv (2018) Preprint
- Matharu N. et al. Science 363, eaau0629 (2019) PubMed
- Sanders S.J. et al. Trends Neurosci. 41, 442-456 (2018) PubMed
- Ben-Shalom R. et al. Biol. Psychiatry 82, 224-232 (2017) PubMed
- Spratt P.W.E. et al. Neuron 103, 673-685 (2019) PubMed
- Rubenstein J.L. and Merzenich M.M. Genes Brain Behav. 2, 255-267 (2003) PubMed
- Tatavarty V. et al. bioRxiv (2018) Preprint
- Serret S. et al. BMC Psychiatry 15, 107 (2015) PubMed
- Miller E.A. et al. bioRxiv (2019) Preprint
- Walker M.P. et al. Neuron 35, 205-211 (2002) PubMed
- Wamsley E.J. et al. Biol. Psychiatry 71, 154-161 (2012) PubMed
- Manoach D. et al. Front. Hum. Neurosci. 8, 762 (2014) PubMed
- Lustenberger C. et al. Curr. Biol. 26, 2127-2136 (2016) PubMed
- Dawson G. et al. Sci. Rep. 8, 17008 (2018) PubMed
- Hong W. et al. Proc. Natl. Acad. Sci. USA 112, E5351-E5360 (2015) PubMed
- Klaus A. et al. Neuron 95, 1171-1180 (2017) PubMed
- Wiltschko A.B. et al. Neuron 88, 1121-1135 (2015) PubMed
- Markowitz J.E. et al. Cell 174, 44-58 (2018) PubMed
- 16p11.2
- Angelman syndrome
- astrocytes
- CRISPR
- E/I balance
- FMR1
- fragile X syndrome
- gene expression
- gene networks
- homeostatic synaptic plasticity
- human research studies
- missense mutations
- mouse models
- organoids
- RNA splicing
- schizophrenia
- SCN2A
- SHANK3
- Simons Searchlight
- Simons Simplex Collection
- sleep
- SPARK
- stem cells
- striatum
- UBE3A
- whole-genome sequencing