
On April 17, 2018, molecular neuroscientists and geneticists gathered at the Simons Foundation for a workshop on proteins associated with chromatin — the complex that packages DNA into a compact structure — and their role in autism spectrum disorder (ASD). Organized by the Simons Foundation Autism Research Initiative (SFARI) and moderated by SFARI senior scientist Alan Packer, the workshop examined recent findings implicating chromatin-associated proteins in the regulation of gene expression, and discussed changes in these proteins that may confer a risk of ASD and other neurodevelopmental disorders.
Why chromatin? Recent studies have shown that a proportion of autism-associated genes, including strong candidate risk factors of the disorder, encode chromatin-associated proteins1 (Table 1). This finding adds to previous research indicating that many autism risk genes regulate synaptic function and, since chromatin-associated proteins regulate gene expression and promote chromatin and DNA modifications, it suggests a role of epigenetic mechanisms in ASD etiology. While these observations may open new avenues for autism research and deepen the understanding of the molecular mechanisms of the disorder, much still remains to be learned about the biology and function of chromatin-associated proteins in brain development. This workshop was intended to provide a platform to begin discussing these questions, while considering translational opportunities and evaluating ways that SFARI could potentially help to move research in this area forward.
Speakers included Schahram Akbarian (Icahn School of Medicine at Mount Sinai), Hongjun Song (University of Pennsylvania), Anne Schaefer (Icahn School of Medicine at Mount Sinai), Zhen Yan (State University of New York at Buffalo), Erica Korb (The Rockefeller University), Stephanie Bielas (University of Michigan Medical School), Nael Nadif Kasri (Radboud University Medical Center) and Stephen Haggarty (Harvard Medical School).
Table 1. Several autism risk genes encode chromatin-associated proteins. SFARI Gene lists over 80 genes that are deemed to confer risk for autism with high confidence or being strong candidates for the disorder. Of these, several are involved in chromatin-related processes. SFARI Gene scoring categories: 1S (high confidence, syndromic), 1 (high confidence), 2 (strong candidate).
-
ADNP 1S Potential transcription factor that may mediate some of the neuroprotective peptide VIP-associated effects involving normal growth and cancer proliferation. ANKRD11 2S A locus that encodes an ankyrin repeat domain-containing protein that inhibits ligand-dependent activation of transcription. ARID1B 1S Encodes an AT-rich DNA interacting domain-containing protein that is part of the SWI/SNF chromatin remodeling complex. ASH1L 1 Encodes a member of the trithorax group of transcriptional activators that functions as a histone methyltransferase. ASXL3 1S A putative Polycomb group protein that maintains transcription repression (likely via the methylation of histones). BAZ2B 2 A member of the bromodomain gene family, which encodes proteins involved in chromatin remodeling. BCL11A 2 Encodes a C2H2 type zinc-finger protein. CHD2 1S DNA helicase, chromatin remodeling factor. CHD8 1S DNA helicase, chromatin remodeling factor. KAT2B 2 Encodes a protein that functions as a histone acetyltransferase (HAT) to promote transcriptional activation and acts as a circadian transcriptional coactivator that enhances the activity of the circadian transcriptional activators. KMT2A 1S Histone methyltransferase that mediates chromatin modifications associated with epigenetic transcriptional activation. KMT2C 2S Histone methyltransferase that methylates ‘Lys-4’ of histone H3, a specific tag for epigenetic transcriptional activation. KMT5B 1 Histone methyltransferase that plays a central role in establishing heterochromatin in pericentric regions. MECP2 2S Encodes a protein that has methylation-dependent transcriptional repressor activity. It is involved in regulation of RNA splicing. POGZ 1S Encodes a zinc finger protein that contains a transposase domain at the C-terminus. It plays a role in mitotic cell cycle progression and is involved in kinetochore assembly and mitotic sister chromatid cohesion. SETD5 1S Encodes a methyltransferase and resides within the critical interval for the 3p25 microdeletion syndrome. SMARCC2 2 Encodes a protein that is part of the SWI/SNF chromatin remodeling complex. SRCAP 2 Encodes the core catalytic component of the multiprotein chromatin-remodeling SRCAP complex. TBL1XR1 2 Encodes a component of both the nuclear receptor corepressor (N-CoR) and histone deacetylase 3 (HDAC3) complexes. It is required for transcriptional activation by a variety of transcription factors.
Structure and function
The first speaker at the workshop, Schahram Akbarian, emphasized the need to examine the three-dimensional genome — the spatial architecture of the genome including scaffolding proteins and organizers — whose regulation is important for neurodevelopment, cognition and behavior2. He argued that the way the genome is packed has implications for neurodevelopmental disorders and described findings from a recent study showing that locus-specific alterations of chromosome structure in the brain after neuronal ablation of the methytransferase Setdb1 led to changes in transcription and gene expression3.
Shifting the focus from structure to function, SFARI investigator Hongjun Song then highlighted the dynamic nature of modifications occurring at the levels of the epigenome as well as the epitranscriptome4 (Figure 1). For instance, he showed that the mRNA modification N6-methyladenosine (m6A) is instrumental for the temporal coordination of cortical neurogenesis and appears implicated in risk for neurodevelopmental and psychiatric disorders such as ASD and schizophrenia5. With a SFARI Research Award, Song now plans to expand this work and further investigate the role of m6A modifications in the regulation of ASD risk genes.
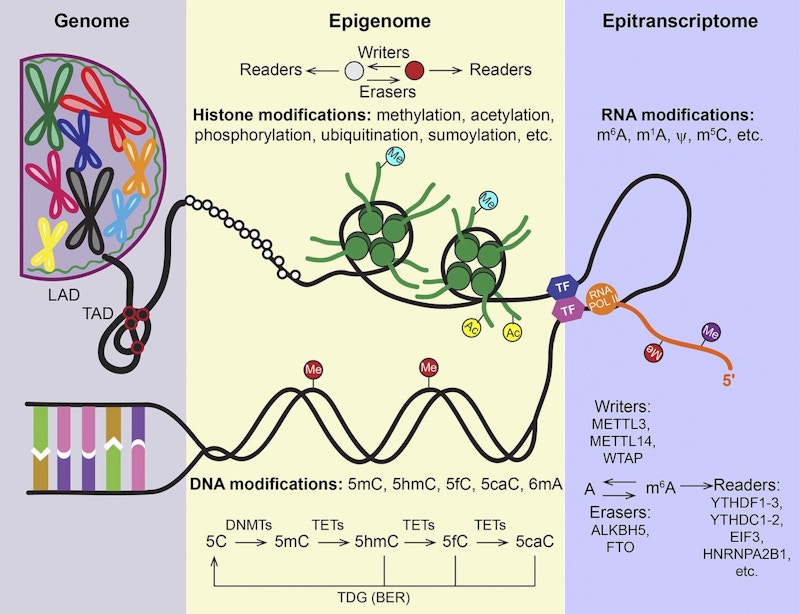
Reversible epigenetic changes?
A number of presentations then discussed whether ASD-related phenotypes associated with epigenetic changes may be reversible. Lending support to this possibility, Anne Schaefer presented two lines of evidence from her mouse-based research. First, she showed that systemic administration of a brain-permeable inhibitor of the bromodomain and extraterminal domain–containing (BET) proteins — which play a role in elongation transcription — disrupted the expression of long genes regulating neuronal differentiation and synaptic activity, including several ASD risk genes7. This pharmacological manipulation also produced a loss of social preference in mice that received the treatment. However, this phenotype was restored several weeks following the end of the treatment, thus suggesting that the epigenetic changes induced in Schaefer’s pharmacological mouse model of ASD were reversible. Second, she provided evidence that pharmacologically induced alterations at the cellular level that are often observed in ASD (e.g., increased numbers of dendritic spines) could be similarly reversed once the treatment was removed.
Zhen Yan then described an epigenetic mechanism-based treatment that rescued ASD-related phenotypes in Shank3-deficient mouse models, by targeting histone deacetylase (HDAC) complexes — proteins that can silence gene expression by changing chromatin structure8. This treatment involved the use of a class I HDAC inhibitor, romidepsin, which is an FDA-approved anticancer drug. In her study, Yan showed that HDAC2 transcription was upregulated in the prefrontal cortex of mice lacking Shank3 and found that this alteration was mediated by a β-catenin-mediated transcriptional mechanism. Crucially, administering small doses of romidepsin to Shank3-deficient mice exerted a prolonged rescue of deficits in social behaviors that are typically observed in Shank3 knockout mice, and normalized the expression and function of synaptic proteins, with no major side effects.
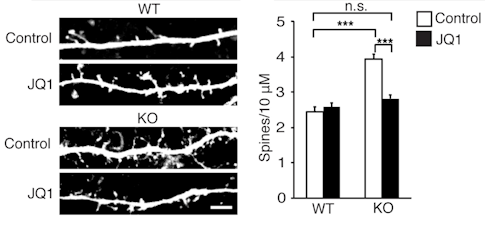
Another example of phenotype reversibility was provided by Erica Korb, a postdoctoral fellow in David Allis’ laboratory, who discussed the role of epigenetic mechanisms in fragile X syndrome, a leading cause of autism that results from the loss of the fragile X mental retardation protein (FMRP). Korb and colleagues found that one target of FMRP, the chromatin-associated protein Brd4, is upregulated in Fmr1 knockout mice9. They then used the inhibitor JQ1 to repress the activity of bromodomain and extraterminal domain–containing (BET) proteins, including Brd4, and found that genes that were upregulated in mutant mice returned to wild-type levels. JQ1 also had several effects at the cellular and behavioral level. Dendritic spine numbers, which are typically increased in fragile X syndrome, decreased to wild-type levels (Figure 2), and social behavioral deficits and repetitive behaviors were also reversed by the treatment.
Chromatin changes and stem cell models
The workshop also considered the role of chromatin changes in the context of other autism-associated neurodevelopmental disorders and the use of human induced pluripotent stem cell (iPSC) models to study the molecular underpinnings and functional consequences of those chromatin alterations.
Stephanie Bielas, who was recently awarded a SFARI grant to investigate transcriptional regulation and fate of neural progenitor cells during corticogenesis, discussed her research on ASXL3, a gene associated with Bainbridge-Ropers Syndrome (BRS) and ASD. She showed that fibroblasts derived from individuals with BRS presented alterations in the monoubiquitination of histone H2A lysine 119, which regulates several chromatin-related processes10, 11.
Another speaker, Nael Nadif Kasri, described abnormal electrophysiological signatures in a mouse model of Kleefstra syndrome12 — a neurodevelopmental disorder comorbid with ASD that results from mutations in a gene, EHMT1, that encodes a chromatin-associated protein inhibiting transcription. Kasri also described preliminary findings in iPSCs derived from individuals with Kleefstra syndrome and opportunities to conduct drug screens for this disorder.
The final speaker, Stephen Haggarty, presented results from his research using iPSCs to study the molecular mechanisms of Pitt-Hopkins syndrome, a rare neurodevelopmental disorder resulting from mutations in the transcription factor TCF4. Haggarty showed that different TCF4 transcripts were either upregulated or downregulated in iPSC-derived neural progenitors and differentiated neurons and provided evidence that the canonical WNT/β-catenin signaling pathway regulates the expression of a subset of those TCF4 transcripts via epigenetic mechanisms involving HDAC complexes13. Treating fibroblasts from individuals affected by Pitt-Hopkins syndrome with WNT signaling modulators and class I HDAC inhibitors, he then found that this pharmacological intervention helped upregulate a subset of TCF4 transcripts, which were lower in those with the disorder relative to typically developing individuals (Figure 3). Based upon these studies, the Haggarty lab conducted a large-scale screen of over 300,000 compounds for novel TCF4 enhancing compounds, leading to the identification of a collection of multiple lead compound series that could restore TCF4 expression in Pitt-Hopkins patient-derived neuronal cells. This research may provide a first step toward understanding the biology of ASD-related neurodevelopmental disorders and developing novel pharmacological treatments.

Concluding remarks
This workshop highlighted the role of chromatin-associated proteins in ASD and related neurodevelopmental disorders, an area of investigation that has been gaining increasing attention in the past few years. While discussing a variety of mechanisms, the speakers emphasized the need for a better understanding of the basic biology of chromatin-associated proteins and other complexes. Also, there was consensus that identifying these proteins’ targets and common signaling pathways would be important to fully understand the impact of chromatin-associated changes on phenotype.
The speakers also emphasized the role of in vitro assays in shedding light on the molecular mechanisms underlying epigenetic changes in these disorders and for providing opportunities to perform pharmacological screens. One key recommendation was to invest in stem cell lines, for instance by establishing libraries of iPSCs, to test the efficacy of potential therapeutic approaches, which are currently lacking in the field. To help support some of these efforts, SFARI has been generating iPSC lines from cells obtained from participants of the Simons Variation in Individuals Project (Simons VIP), who carry known copy number variants and single gene mutations associated with autism and other neurodevelopmental disorders. iPSC lines from individuals with 16p11.2 deletions and duplications are currently available to researchers via SFARI Base. iPSC lines from individuals with mutations in at least 15 different single genes are expected to be available for ordering in the near future.
References
- De Rubeis S. et al. Nature 515, 209-215 (2014) PubMed
- Rajarajan P. et al. Nat. Rev. Neurosci. 17, 681-691 (2016) PubMed
- Jiang Y. et al. Nat. Genet. 49, 1239-1250 (2017) PubMed
- Yoon K.J. et al. J. Cell Biol. 217, 1901-1914 (2018) PubMed
- Yoon K.J. et al. Cell 171, 877-889 (2017) PubMed
- Huang S. et al. Semin. Cell Dev. Biol. 20, 869-876 (2009) PubMed
- Sullivan J.M. et al. J. Exp. Med. 212, 1771-1781 (2015) PubMed
- Qin L. et al. Nat. Neurosci. 21, 564-575 (2018) PubMed
- Korb E. et al. Cell 170, 1209-1223 (2017) PubMed
- Srivastava A. et al. Hum. Mol. Genet. 25, 597-608 (2016) PubMed
- Srivastava A. et al. Trends Genet. 33, 566-578 (2017) PubMed
- Benevento M. et al. Neuron 91, 341-355 (2016) PubMed
- Henning K.M. et al. Mol. Neuropsychiatry 3, 53-71 (2017) PubMed